Transient receptor potential (TRP) ion channels are gated by diverse intra- and extracellular stimuli leading to cation inflow (Na+, Ca2+) regulating many cellular processes and initiating organismic somatosensation. *
Structures of most TRP channels have been solved. However, structural and sequence analysis showed that ~30% of the TRP channel sequences, mainly the N- and C-termini, are intrinsically disordered regions (IDRs). Unfortunately, very little is known about IDR ‘structure’, dynamics and function, though it has been shown that they are essential for native channel function. *
In the article “Intrinsically disordered regions in TRPV2 mediate protein-protein interactions”, Raghavendar R. Sanganna Gari, Grigory Tagiltsev, Ruth A. Pumroy, Yining Jiang, Martin Blackledge, Vera Y. Moiseenkova-Bell and Simon Scheuring imaged TRPV2 channels in membranes using high-speed atomic force microscopy (HS-AFM). *
The dynamic single molecule imaging capability of HS-AFM allowed the authors to visualize IDRs and revealed that N-terminal IDRs were involved in intermolecular interactions. Their work provides evidence about the ‘structure’ of the TRPV2 IDRs, and that the IDRs may mediate protein-protein interactions. *
In total, 1.5 µl of the TRPV2 reconstituted vesicles were deposited on a 1.5-mm2 freshly cleaved mica surface, which was glued with epoxy to the quartz sample stage. After 20–30 min incubation, the sample was gently rinsed with imaging buffer (20 mM Hepes, pH 8.0, 150 mM NaCl) and mounted in the HS-AFM fluid cell. All images in this study were taken using a HS-AFM operated in amplitude modulation mode using optimized scan and feedback parameters and lab-built amplitude detectors and free amplitude stabilizers. *
Short (8 µm) cantilevers (NanoWorld Ultra-Short Cantilevers for High-Speed AFM USC-F1.2-k0.15) with nominal spring constant of 0.15 N/m, resonance frequency of 0.6 MHz, and a quality factor of ∼1.5 in liquid were used. AFM probes were sharpened using oxygen plasma etching to obtain better resolution. *
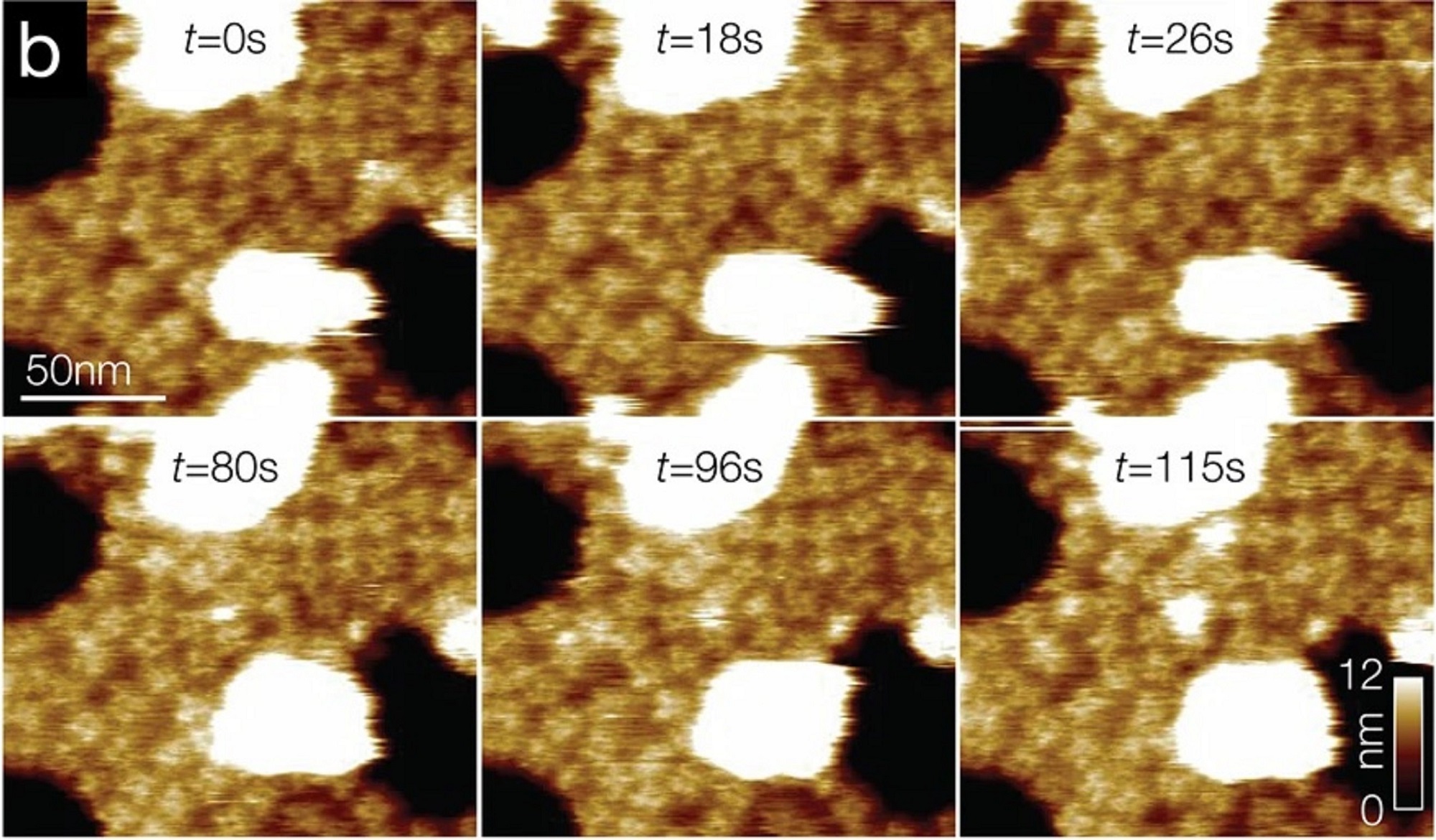
TRPV2 reconstitution for HS-AFM analysis.
b Overview HS-AFM images (Supplementary Movie 1) of TRPV2 (windmill-shaped molecules) in soy polar lipid membranes on mica (dark background areas). False color scale: 0–9 nm. The white oversaturated areas have a height of ~26 nm and represent likely non-ruptured small vesicles.
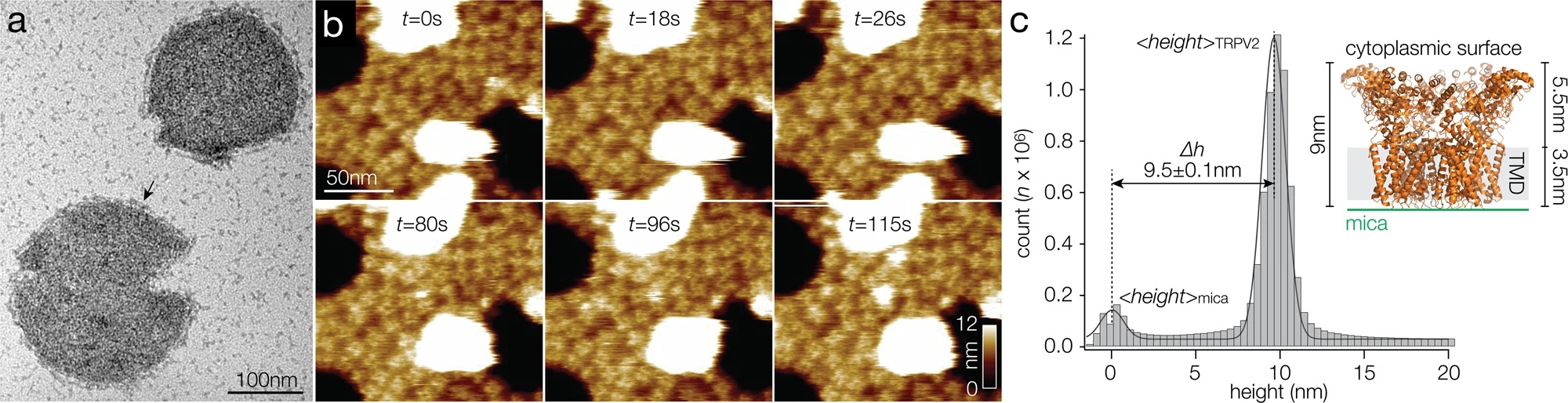
TRPV2 reconstitution for HS-AFM analysis.
a Negative-stain EM of TRPV2 reconstituted into soy polar lipids at a lipid-to-protein ratio of 0.7. Protruding features (arrow) at the vesicle periphery and the strong contrast of the proteins in the vesicle in the negative-stain EM are indicative of inside-out reconstitution of the TRPV2 channels with the large cytoplasmic domains exposed to the outside of the vesicle. b Overview HS-AFM images (Supplementary Movie 1) of TRPV2 (windmill-shaped molecules) in soy polar lipid membranes on mica (dark background areas). False color scale: 0–9 nm. The white oversaturated areas have a height of ~26 nm and represent likely non-ruptured small vesicles. c Height distribution of TRPV2 above mica from (b). TRPV2 has a full height of 9.5 ± 0.1 nm above mica, in good agreement with the TRPV2 cryo-EM structure. Inset: Cryo-EM structure PDB 6U84 shown with the intracellular side up (as imaged by HS-AFM), membrane indicated in light gray.
*Raghavendar R. Sanganna Gari, Grigory Tagiltsev, Ruth A. Pumroy, Yining Jiang, Martin Blackledge, Vera Y. Moiseenkova-Bell and Simon Scheuring
Intrinsically disordered regions in TRPV2 mediate protein-protein interactions
Communications Biology volume 6, Article number: 966 (2023)
DOI: https://doi.org/10.1038/s42003-023-05343-7
Please follow this external link to read the full article: https://rdcu.be/dnNba
The article “Phosphorylation of phase-separated p62 bodies by ULK1 activates a redox-independent stress response” by Raghavendar R. Sanganna Gari, Grigory Tagiltsev, Ruth A. Pumroy, Yining Jiang, Martin Blackledge, Vera Y. Moiseenkova-Bell and Simon Scheuring is licensed under a Creative Commons Attribution 4.0 International License, which permits use, sharing, adaptation, distribution and reproduction in any medium or format, as long as you give appropriate credit to the original author(s) and the source, provide a link to the Creative Commons license, and indicate if changes were made. The images or other third-party material in this article are included in the article’s Creative Commons license, unless indicated otherwise in a credit line to the material. If material is not included in the article’s Creative Commons license and your intended use is not permitted by statutory regulation or exceeds the permitted use, you will need to obtain permission directly from the copyright holder. To view a copy of this license, visit https://creativecommons.org/licenses/by/4.0/.