The Endosomal Sorting Complex Required for Transport-III (ESCRT-III) is part of a conserved membrane remodeling machine. ESCRT-III employs polymer formation to catalyze inside-out membrane fission processes in a large variety of cellular processes, including budding of endosomal vesicles and enveloped viruses, cytokinesis, nuclear envelope reformation, plasma membrane repair, exosome formation, neuron pruning, dendritic spine maintenance, and preperoxisomal vesicle biogenesis.*
How membrane shape influences ESCRT-III polymerization and how ESCRT-III shapes membranes is yet unclear.*
In the article “Human ESCRT-III polymers assemble on positively curved membranes and induce helical membrane tube formation” Aurélie Bertin, Nicola de Franceschi, Eugenio de la Mora, Sourav Maity, Maryam Alqabandi, Nolwen Miguet, Aurélie di Cicco, Wouter H. Roos, Stéphanie Mangenot, Winfried Weissenhorn and Patricia Bassereau describe how human core ESCRT-III proteins, CHMP4B, CHMP2A, CHMP2B and CHMP3 are used to address this issue in vitro by combining membrane nanotube pulling experiments, cryo-electron tomography and Atomic Force Microscopy.*
The authors show that CHMP4B filaments preferentially bind to flat membranes or to tubes with positive mean curvature.*
The results presented in the article cited above underline the versatile membrane remodeling activity of ESCRT-III that may be a general feature required for cellular membrane remodeling processes.*
The authors provide novel insight on how mechanics and geometry of the membrane and of ESCRT-III assemblies can generate forces to shape a membrane neck.*
NanoWorld Ultra-Short AFM Cantilevers USC-F1.2-k0.15 were used for the High-speed Atomic Force Microscopy ( HS-AFM ) experiments presented in this article.*
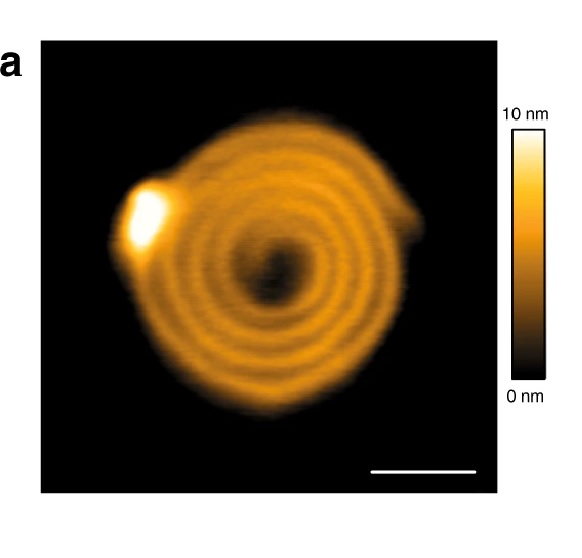
CHMP4-ΔC flattens LUVs and binds preferentially to flat membranes or to membranes with a positive mean curvature.
1a CHMP4B-ΔC spirals observed by HS-AFM on a lipid bilayer. Scale bar: 50 nm.
Please refer to the full article for the complete figure: https://rdcu.be/b5rOe
*Aurélie Bertin, Nicola de Franceschi, Eugenio de la Mora, Sourav Maity, Maryam Alqabandi, Nolwen Miguet, Aurélie di Cicco, Wouter H. Roos, Stéphanie Mangenot, Winfried Weissenhorn and Patricia Bassereau
Human ESCRT-III polymers assemble on positively curved membranes and induce helical membrane tube formation
Nature Communications volume 11, Article number: 2663 (2020)
DOI: https://doi.org/10.1038/s41467-020-16368-5
Please follow this external link to read the full article: https://rdcu.be/b5rOe
Open Access The article “ Human ESCRT-III polymers assemble on positively curved membranes and induce helical membrane tube formation “ by Aurélie Bertin, Nicola de Franceschi, Eugenio de la Mora, Sourav Maity, Maryam Alqabandi, Nolwen Miguet, Aurélie di Cicco, Wouter H. Roos, Stéphanie Mangenot, Winfried Weissenhorn and Patricia Bassereau is licensed under a Creative Commons Attribution 4.0 International License, which permits use, sharing, adaptation, distribution and reproduction in any medium or format, as long as you give appropriate credit to the original author(s) and the source, provide a link to the Creative Commons license, and indicate if changes were made. The images or other third party material in this article are included in the article’s Creative Commons license, unless indicated otherwise in a credit line to the material. If material is not included in the article’s Creative Commons license and your intended use is not permitted by statutory regulation or exceeds the permitted use, you will need to obtain permission directly from the copyright holder. To view a copy of this license, visit http://creativecommons.org/licenses/by/4.0/.