Boron doped diamond (BDD) has great potential in electrical, and electrochemical sensing applications. The growth parameters, substrates, and synthesis method play a vital role in the preparation of semiconducting BDD to metallic BDD. Doping of other elements along with boron (B) into diamond demonstrated improved efficacy of B doping and exceptional properties.*
In the article “Influence of B/N co-doping on electrical and photoluminescence properties of CVD grown homoepitaxial diamond films” Srinivasu Kunuku, Mateusz Ficek, Aleksandra Wieloszynska, Magdalena Tamulewicz-Szwajkowska, Krzysztof Gajewski, Miroslaw Sawczak, Aneta Lewkowicz, Jacek Ryl, Tedor Gotszalk and Robert Bogdanowicz describe how B and nitrogen (N) co-doped diamond has been synthesized on single crystalline diamond (SCD) IIa and SCD Ib substrates in a microwave plasma-assisted chemical vapor deposition process.*
The surface topography of the CVD diamond layers was investigated using atomic force microscopy (AFM), and Kelvin probe force microscopy (KPFM) was employed to measure the contact potential difference (CPD) to calculate the work function of these CVD diamond layers.*
Atomic force microscopy topography depicted the flat and smooth surface with low surface roughness for low B doping, whereas surface features like hillock structures and un-epitaxial diamond crystals with high surface roughness were observed for high B doping concentrations. KPFM measurements revealed that the work function (4.74–4.94 eV) has not varied significantly for CVD diamond synthesized with different B/C concentrations.*
NanoWorld ARROW-EFM conductive platinumirdidium5 coated AFM probes with a typical spring constant of 2.8 N/m and a typical resonant frequency of 75 kHz were used.*
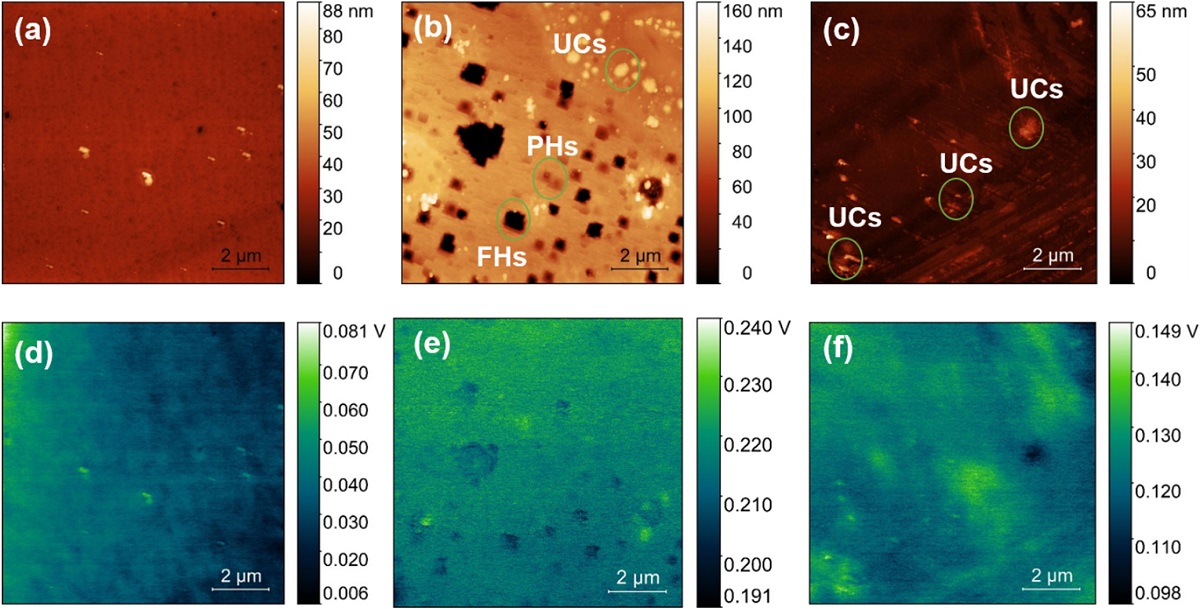
AFM topography of B/N co-doped CVD diamond on (with fixed N/C = 0.02) SCD IIa; (a) B/C ∼ 2500 ppm (b) B/C ∼ 5000 ppm (c) B/C ∼ 7500 ppm, and KPFM CPD images of B/N co-doped CVD diamond (with fixed N/C = 0.02) on SCD IIa; (d) B/C ∼ 2500 ppm (e) B/C ∼ 5000 ppm (f) B/C ∼ 7500 ppm.
*Srinivasu Kunuku, Mateusz Ficek, Aleksandra Wieloszynska, Magdalena Tamulewicz-Szwajkowska, Krzysztof Gajewski, Miroslaw Sawczak, Aneta Lewkowicz, Jacek Ryl, Tedor Gotszalk and Robert Bogdanowicz
Influence of B/N co-doping on electrical and photoluminescence properties of CVD grown homoepitaxial diamond films
Nanotechnology (2022), 33 125603
DOI: https://doi.org/10.1088/1361-6528/ac4130
Please follow this external link to read the full article: https://doi.org/10.1088/1361-6528/ac4130
Open Access The article “Influence of B/N co-doping on electrical and photoluminescence properties of CVD grown homoepitaxial diamond films” by Srinivasu Kunuku, Mateusz Ficek, Aleksandra Wieloszynska, Magdalena Tamulewicz-Szwajkowska, Krzysztof Gajewski, Miroslaw Sawczak, Aneta Lewkowicz, Jacek Ryl, Tedor Gotszalk and Robert Bogdanowicz is licensed under a Creative Commons Attribution 4.0 International License, which permits use, sharing, adaptation, distribution and reproduction in any medium or format, as long as you give appropriate credit to the original author(s) and the source, provide a link to the Creative Commons license, and indicate if changes were made. The images or other third party material in this article are included in the article’s Creative Commons license, unless indicated otherwise in a credit line to the material. If material is not included in the article’s Creative Commons license and your intended use is not permitted by statutory regulation or exceeds the permitted use, you will need to obtain permission directly from the copyright holder. To view a copy of this license, visit http://creativecommons.org/licenses/by/4.0/.