Anisotropic materials with oppositely signed dielectric tensors support hyperbolic polaritons, displaying enhanced electromagnetic localization and directional energy flow. *
However, the most reported hyperbolic phonon polaritons are difficult to apply for active electro-optical modulations and optoelectronic devices. *
In the nature communications letter “Manipulating hyperbolic transient plasmons in a layered semiconductor”, Rao Fu, Yusong Qu, Mengfei Xue, Xinghui Liu, Shengyao Chen, Yongqian Zhao, Runkun Chen, Boxuan Li, Hongming Weng, Qian Liu, Qing Dai and Jianing Chen report a dynamic topological plasmonic dispersion transition in black phosphorus (BP) via photo-induced carrier injection, i.e., transforming the iso-frequency contour from a pristine ellipsoid to a non-equilibrium hyperboloid. *
They introduce a promising approach to optically manipulate robust transient hyperbolic plasmons in the layered semiconductor black phosphorus using a dedicated ultrafast nanoscopy scheme. Optical pumping allows the BP’s IFCs to topologically transit from the pristine ellipsoid to the non-equilibrium hyperboloid, exhibiting exotic non-equilibrium hyperbolic plasmon properties, such as the optically tunable plasmonic dispersion and the coexistence of different transient plasmonic modes. *
Their work also demonstrates the peculiar transient plasmonic properties of the studied layered semiconductor, such as the ultrafast transition, low propagation losses, efficient optical emission from the black phosphorus’s edges, and the characterization of different transient plasmon modes. *
The results that Rao Fu et al. present may be relevant for the development of future optoelectronic applications. *
NanoWorld® ARROW-NCPt AFM probes with a Pt/Ir coating were used for the characterization with ultrafast nanoscopy. The pump and probe pulses were spatially overlapped on the Platinum/Iridium coated Arrow probe through a parabolic mirror of a commercial scattering-type scanning near-field optical microscope. *
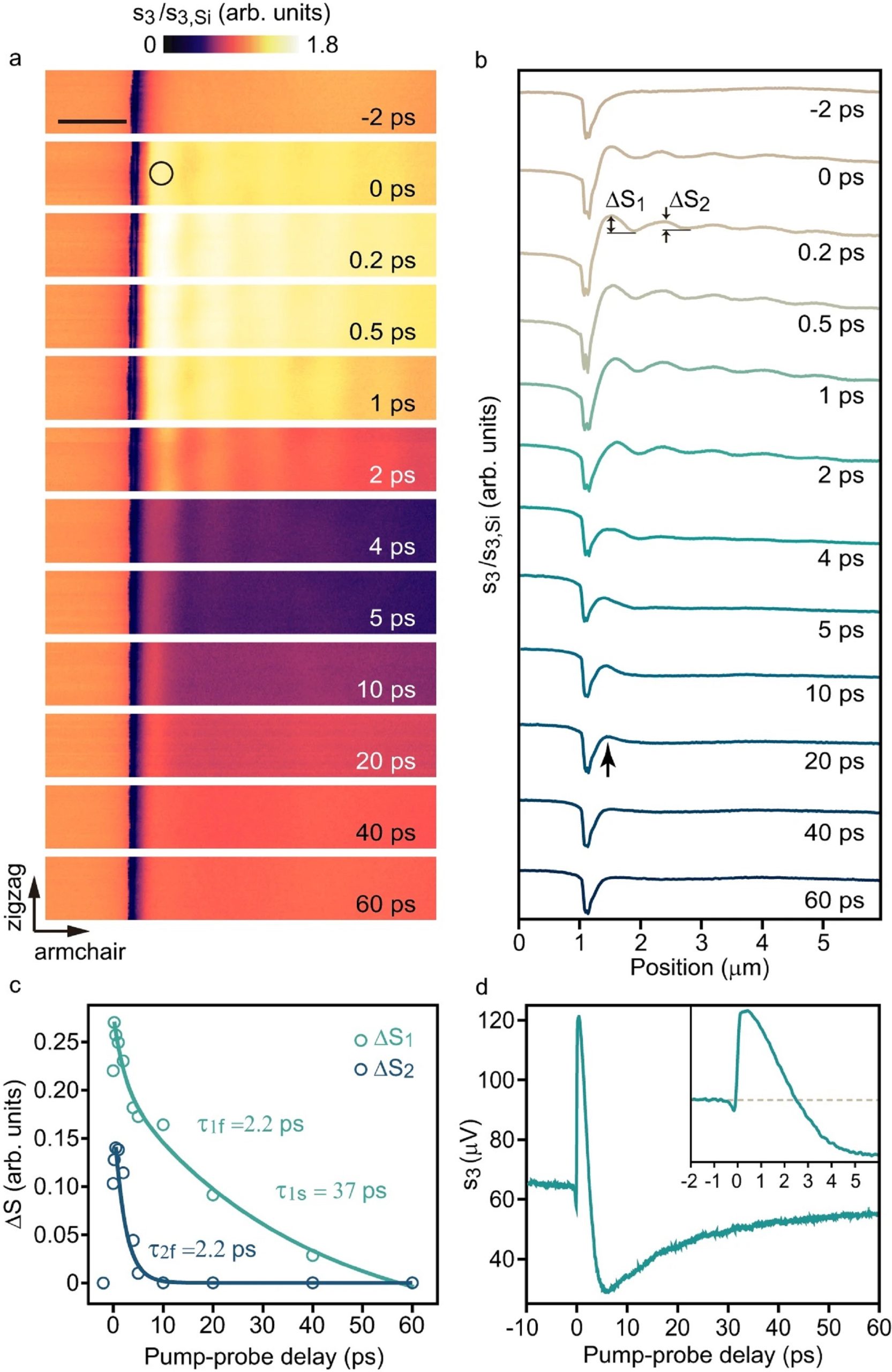
Dynamic analysis of the transient plasmons.
a Normalized near-field amplitude s3/s3,Si of a 280-nm-thick BP slab for twelve delay times τ. Scale bar, 1 µm. b Near-field amplitude curves for the corresponding twelve different delay times τ in a. c Dynamics of the relative near-field intensity of the first (∆S1) and the second bright strip (∆S2) in b. Opened circles are the experimental data, and solid lines are bi-exponential fitting for ∆S1 and exponential fitting for ∆S2, respectively. d Dynamics of the near-field amplitude s3 from the black circle in a. The inset displays the s3 at τ = −2 to 6 ps, and the dashed line marks the s3 level of the pristine state.
*Rao Fu, Yusong Qu, Mengfei Xue, Xinghui Liu, Shengyao Chen, Yongqian Zhao, Runkun Chen, Boxuan Li, Hongming Weng, Qian Liu, Qing Dai and Jianing Chen
Manipulating hyperbolic transient plasmons in a layered semiconductor
Nature Communications volume 15, Article number: 709 (2024)
DOI: https://doi.org/10.1038/s41467-024-44971-3
Please follow this external link to read the full article: https://rdcu.be/dBD85
The article “Manipulating hyperbolic transient plasmons in a layered semiconductor” by Rao Fu, Yusong Qu, Mengfei Xue, Xinghui Liu, Shengyao Chen, Yongqian Zhao, Runkun Chen, Boxuan Li, Hongming Weng, Qian Liu, Qing Dai and Jianing Chen is licensed under a Creative Commons Attribution 4.0 International License, which permits use, sharing, adaptation, distribution and reproduction in any medium or format, as long as you give appropriate credit to the original author(s) and the source, provide a link to the Creative Commons license, and indicate if changes were made. The images or other third-party material in this article are included in the article’s Creative Commons license, unless indicated otherwise in a credit line to the material. If material is not included in the article’s Creative Commons license and your intended use is not permitted by statutory regulation or exceeds the permitted use, you will need to obtain permission directly from the copyright holder. To view a copy of this license, visit https://creativecommons.org/licenses/by/4.0/.