The behaviour of ions at solid–liquid interfaces underpins countless phenomena, from the conduction of nervous impulses to charge transfer in solar cells. In most cases, ions do not operate as isolated entities, but in conjunction with neighbouring ions and the surrounding solution. In aqueous solutions, recent studies suggest the existence of group dynamics through water-mediated clusters but results allowing direct tracking of ionic domains with atomic precision are scarce. *
Atomic force microscopy (AFM) can image single ions adsorbed at various solid–liquid interfaces. One of the main advantages of the technique is its ability to probe individual ions in-situ but with local contextual information about the interface over tens of nanometres at the point of measurement.*
High-speed atomic force microscopy (HS-AFM) operates similarly to standard AFM but with enhanced temporal resolution and can capture images at video rate, making it possible to track many molecular processes in real-time.*
In the article “Real-time tracking of ionic nano-domains under shear flow” Clodomiro Cafolla and Kislon Voïtchovsky use high-speed atomic force microscopy to track the evolution of Rb+, K+, Na+ and Ca2+ nano-domains containing 20 to 120 ions adsorbed at the surface of mica in aqueous solution. The interface is exposed to a shear flow able to influence the lateral motion of single ions and clusters. *
They report the achievement of single-ion spatial resolution with ~ 2 s temporal resolution.*
During the measurements, the AFM cantilever and the sample were fully immersed in the aqueous ionic solution of interest. The AFM probes used were NanoWorld Arrow-UHF ultra-high frequency AFM cantilevers. *
The results presented in the article provide some quantitative insights into the relationship between single ion properties and group dynamics at the solid–liquid interface in the presence of a microscale shear flow, with potential technological applications from manufacturing biomedical devices to enhancing the performance of aqueous ion-batteries.*
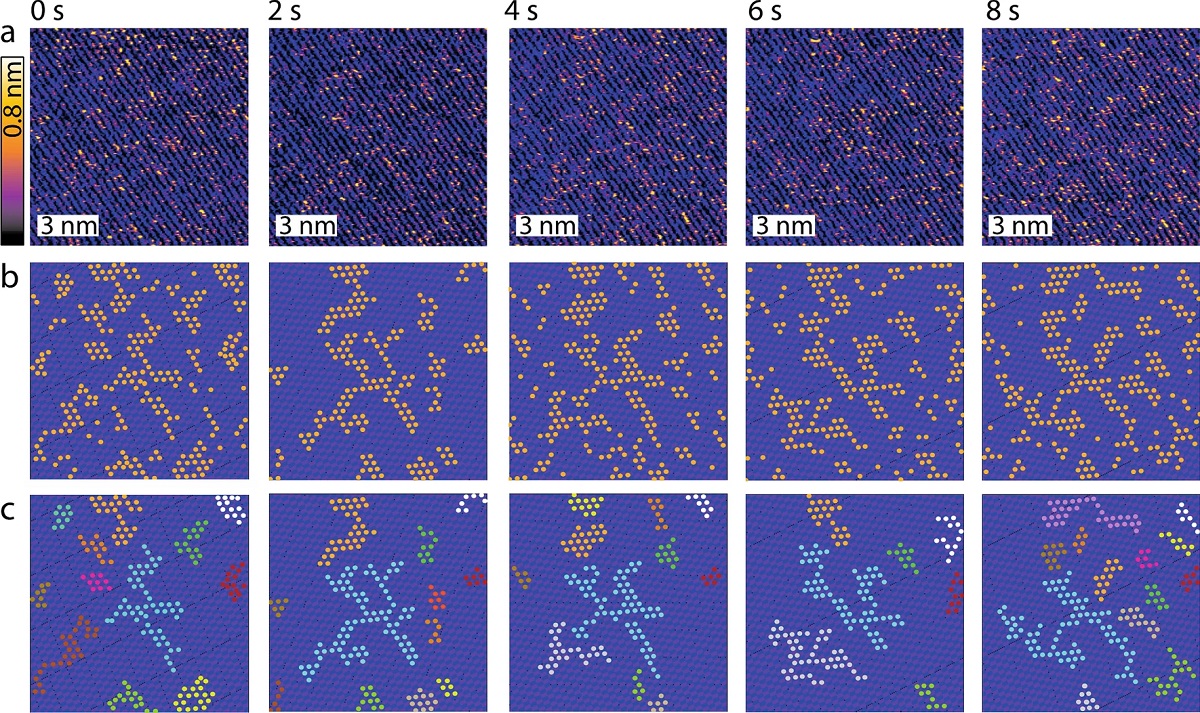
Example of time evolution for adsorbed Rb+ ions at the mica-water interface in the presence of a shear flow. (a) A time-lapse sequence shows consecutive high-resolution HS-AFM topographical images of Rb+ ions at the interface between mica and a 5 mM RbCl aqueous solution. Rb+ ions appear as bright orange-yellow protrusions standing taller than the mica surface (purple-black). Periodic rows and larger domains are clearly visible as well as singly adsorbed rubidium ions. (b) Representative image analysis highlighting Rb+ ions as orange markers (obtained by thresholding) and the idealised underlying lattice derived by inverse Fourier transform of the filtered power spectrum in each image of (a) (see ESI Section 3 for details on the procedure). (c) The algorithm automatically associates neighbouring ions (within distances < 0.52 nm) to the same cluster. Domains smaller than 5 ions are discarded here. The different clusters derived in each image are highlighted using different colours, keeping for each cluster the same colour over time. The cyan-coloured cluster offers a good example of temporal evolution. The scale bars in (a–c) represent 3 nm and the z-scale in (a) corresponds to 0.8 nm.
*Clodomiro Cafolla and Kislon Voïtchovsky
Real-time tracking of ionic nano-domains under shear flow
Nature Scientific Reports volume 11, Article number: 19540 (2021)
DOI: https://doi.org/10.1038/s41598-021-98137-y
Please follow this external link to read the full article: https://rdcu.be/cPRBu
Open Access The article “Real-time tracking of ionic nano-domains under shear flow” by Clodomiro Cafolla and Kislon Voïtchovsky is licensed under a Creative Commons Attribution 4.0 International License, which permits use, sharing, adaptation, distribution and reproduction in any medium or format, as long as you give appropriate credit to the original author(s) and the source, provide a link to the Creative Commons license, and indicate if changes were made. The images or other third party material in this article are included in the article’s Creative Commons license, unless indicated otherwise in a credit line to the material. If material is not included in the article’s Creative Commons license and your intended use is not permitted by statutory regulation or exceeds the permitted use, you will need to obtain permission directly from the copyright holder. To view a copy of this license, visit http://creativecommons.org/licenses/by/4.0/.